On 9 October 2022, a small depression developed in the south Pacific, near Tonga, and in subsequent days this moved towards Antarctica. In so doing it developed explosively to become, so far as we can tell, the deepest extratropical cyclone of modern times. The system’s central pressure fell by over 100 hPa to reach a minimum around 900 hPa at 06 UTC on the 17th, near the edge of the Bellingshausen Sea, and close to the tiny Norwegian dependency of Peter I island. Accordingly, we name this the ‘Peter I storm’ (Peter-the-first). Our primary data source for this study is ECMWF’s ERA5 reanalysis, as low surface observation density in the areas concerned precludes observation-based analysis.
Evolution
The ERA5-based cyclone track figure shows that following genesis in the tropics, the cyclone spent a day or so (10 October) around 23°S, before progressing slowly south-southeastwards on the 11th and 12th, during which time central pressure changed little. On 13th, the low accelerated and turned towards the east-southeast, near 35°S. In tandem, isobars elongated markedly along this track orientation, with renewed development taking place at the eastern end, causing an apparent track jump between 06 and 12 UTC on the 13th. Such an evolutionary feature is not uncommon in the extratropical North Atlantic, when cyclones enter the core of the main extratropical jet. The forward development is referred to as a warm front wave. In the current case, the warm front wave then started deepening and turning poleward, also in a way that is very reminiscent of North Atlantic cyclones. As it approached the Antarctic sea ice edge on the 16th, deepening rates peaked: the maximum 6 h fall was 19 hPa. Part of the Peter I storm’s pressure fall can be attributed to migration into a broadscale annulus of low pressure ‘cyclone debris’ that commonly encircles Antarctica (e.g. see track figure). The lowest pressure value (900.7 hPa) occurred over sea ice, with a coincident near-surface model temperature around –10°C. In the next three days, the cyclone filled slowly and moved slowly in a loop, becoming part of the ‘debris’ and finally losing its identity on the 20th. This 10‑day life cycle is much longer than is typically seen over the North Pacific and North Atlantic, though there are cases of (much less deep) Arctic cyclones lasting as long, particularly in summer. Even if the storm ended up being very cold at its core, latent heat input attributable to its tropical origins may have helped the pressure fall so low.
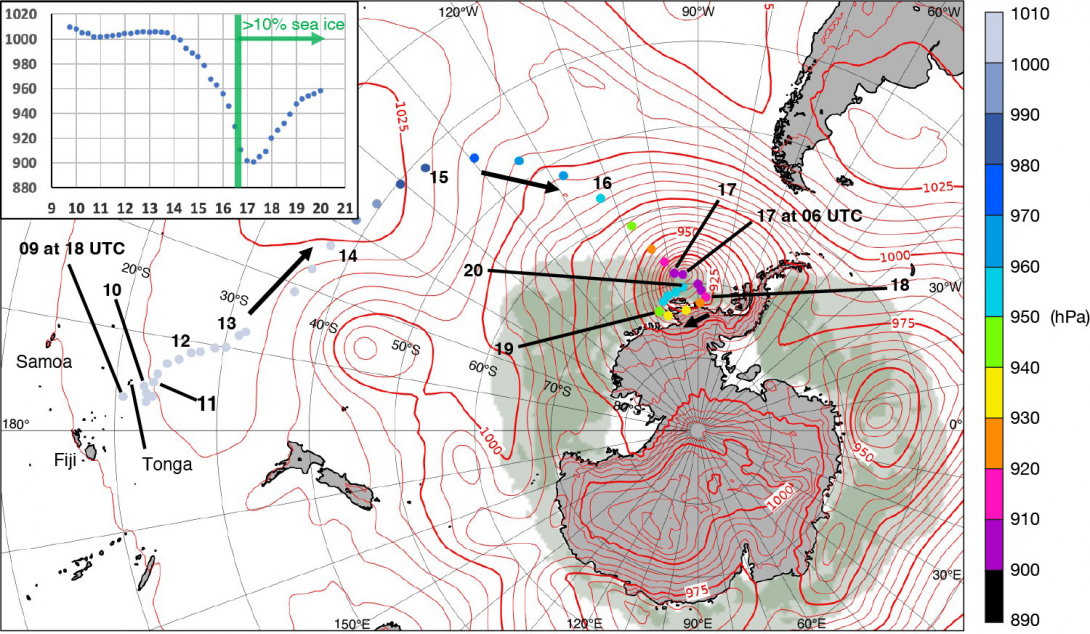
Forecasts
From six days before the event, ECMWF high-resolution forecasts (HRES) were remarkably consistent in predicting a very deep low in the right general area. Pressure minima were generally in the range 895–910 hPa. This reiterates that modern-day models are perfectly capable of producing realistic forecasts of never-before-seen conditions if the initial conditions are conducive. Note also that one would expect a slightly lower minimum in HRES than in ERA5, due to its higher horizontal resolution (9 km versus 31 km).
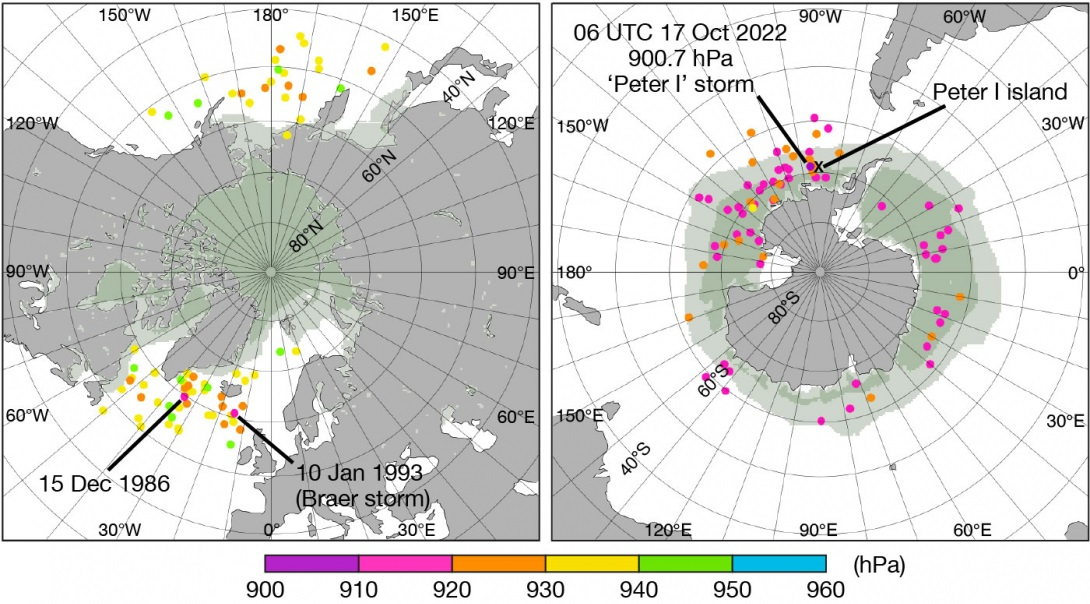
Climatological context and physical considerations
Circumpolar plots of extreme annual surface pressure minima, for the extratropics, highlight that the southern hemisphere consistently experiences lower values than the northern hemisphere. This can be for a variety of reasons, with the very different land–sea distributions being one. The southern hemisphere sequence also shows how the 2022 event really stands out, with pressure values about 10 hPa lower than anything else in the 1950–2021 period. This gives us some confidence that the Peter I storm was the extreme event of modern times, even if a dearth of surface pressure observations reduces our confidence in the absolute value.

It is also interesting that the vast majority of the southern hemisphere annual minima appear to have been over sea ice. They also coincide with months when sea ice is most extensive (maximum extent tends to be in mid–late September). In the northern hemisphere virtually all the annual minima are, meanwhile, over open sea water, with their peak occurrence tending to be closer to the coldest part of the year. These remarks probably have physical significance. The frictional retardation of lower tropospheric flow is less over sea ice than over stormy seas, since sea ice typically has a lower surface roughness. Indeed, over oceans there are destructive feedbacks, whereby the ocean wave growth that coincides with cyclone deepening and attendant strengthening winds ordinarily increases surface roughness. As friction tends to fill cyclones, if other things are equal greater roughness would favour lower deepening rates and higher central pressures.
On the other hand, due to lack of experimental evidence there is considerable uncertainty in roughness lengths over sea ice, particularly if the ice has been distorted or broken up by wave action and strong winds, and this coupled with the low observation density could have conspired to make ERA5 cyclones over sea ice deeper (or indeed shallower) than they should be. This is an area where more research is warranted.
Relative to the ERA5 October climatology for 1990–2020, sea ice cover for the current storm (see track plot) was depleted west of the Antarctic Peninsula, out to about 100°W, and then more extensive beyond that; these anomalies may also have physical and/or dynamical relevance for the surface pressure evolution.
Finally, even though the Peter I storm was a standout event, the time sequence graphs do not seem to provide evidence of any long-term trends in these particular extremes.